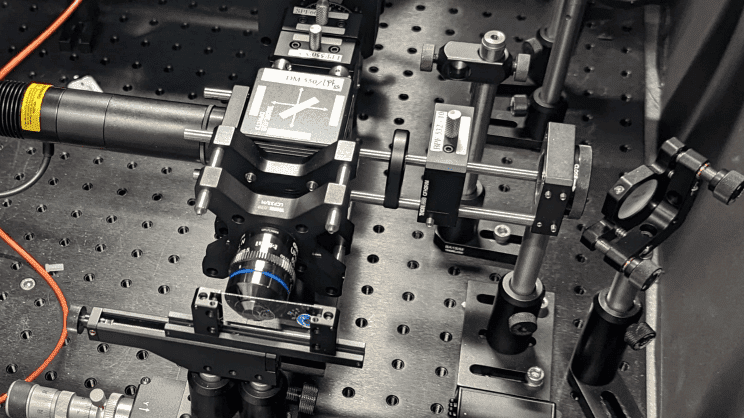
Dr. Sina Saravi leads a junior group at the Institute of Applied Physics (IAP) Jena, thanks to a funding from the Carl-Zeiss Foundation. The group focuses on the fundamental research and experimental development of all-optical diffractive neural networks, specially by utilizing the unique capabilities of nanostructured metasurfaces as an implementation platform. Our target system, namely a Diffractive Deep Neural Network (D^2NN), will be a type of optical computer, made from consecutive layers of diffractive optical elements (such as metasurfaces), in an architecture similar to a deep neural network. Through a joint development of machine-learning algorithms and design of nanostructured optical elements, we aim to design and experimentally realize intelligent optical systems, that can act as an image recognition system or a complex image processor, which receives its input directly in the form of optical waves and analyzes this information all optically. Such a neuromorphic photonic system can be used in machine-vision applications, which specially require extremely fast response times and low energy consumption, which are two of the main current challenges facing the conventional implementation of deep neural networks using electronic systems.
Utilizing linear and nonlinear nanostructured metasurfaces, with specially engineered responses, could open a way towards overcoming some of the challenges in implementation and also unlock the full potential of such optical neural networks. Some of the main challenges are: realizing efficient all-optical nonlinear activation elements, practical realization of a multi-layer system, and the effective use of the various physical degrees of freedom of light in a neural network architecture. Hence, our research has a strong focus on investigating the optical response and realization of nano/micro-structured linear and nonlinear diffractive optical elements, specially metasurfaces. We also develop machine-learning algorithms that are compatible to the underlying physics and can be used in training of the design parameters of such optical systems.
In addition to investigating nanostructured/nanoscale photonic systems for their application in classical optical information processing (namely the optical neural networks), we also investigate such systems for their applications in quantum optical information processing. In particular, we investigate the theoretical formulation, design, and implementation of novel nonlinear sources of quantum light (e.g. single-photon states, entangled photon-pair states, and squeezed-light states) in nonlinear metasurfaces and other types of nano/micro-structured nonlinear systems that are hybridized with 2D materials, atomic/atom-like systems, or nanoparticles.
The optimal realization of D²NNs also requires a deep understanding of, not only light generation in nonlinear diffractive optical elements, but also the free-space propagation, interaction with further diffractive elements, and imaging of this light in the non-paraxial limit. In this regard, Dr. Saravi’s team and coworkers have recently investigated these physics in the context of quantum imaging, where quantum light is generated in a sub-wavelength-thin nonlinear slab, which either interacts with a near-field absorptive nanoparticle [4] or far-field objects in a nonlinear interferometer [5]. The focus of these investigations was to predict the image of the object at the end of the imaging system, specially to find the limit of spatial resolution achievable in such nonlinear quantum systems that involve light beams of two different wavelengths (one photon interacting with the object and the other photon in the pair, with a different wavelength, detected at the camera for imaging). In the case of the far-field (near-field) interactions with the object, we have found that the resolution is limited by the diffraction limit of the longer-wavelength (shorter-wavelength) photon in the pair. To treat these nonlinear imaging problems at their limit, special attention was given to non-paraxial formulation of the light generation and propagation. These results set the stage for finding the ultimate capability of our D²NNs in terms of layer thicknesses and pixel sizes.
Furthermore, Dr. Saravi and his team pursue the design of novel sources of quantum light, where nonlinear sources of photon pairs/squeezed light are hybridized with atomic/atom-like systems, where the presence of the atom-like system fundamentally modifies the dynamics of quantum light generation in such systems [6]. Recently, we have theoretically shown that the statistics of quantum light generation in a nonlinear cavity can be completely modified by hybridizing it with a 2-level atomic/atom-like system [7], reaching a regime of quantum-light generation that is fundamentally unattainable in nonlinear sources. More specifically, we have shown that with a properly chosen sequence of pump pulses in such a hybrid system, we can dramatically increase the probability of generating photon-number Fock states. Moreover, we also develop theoretical formulations, based on the Green’s function method, to study the dynamics of high-gain parametric down-conversion processes, which is capable of treating nanostructured systems with inherently complex dispersive and lossy properties [8].
[1] S. Liu et al., Nano Lett.16, 5426 (2016). |